Overview
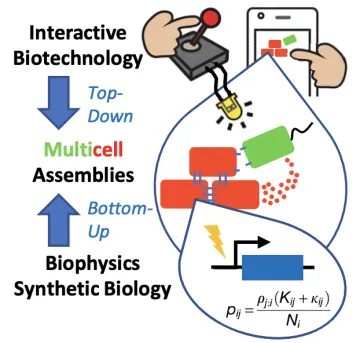
Multicellularity enables organisms and symbiotic systems to achieve complex tasks through division of labor among cells. Such systems transcend current man-made materials and electronics in many ways, e.g., they synthesize chemicals, generate active physical forms, and self-replicate. The ability to harness these features promises significant impact for research (build-to-understand methodology), manufacturing (smart materials), health (tissue engineering), chemistry (pathway modularization), ecology (bioremediation), biodesign (art), and more. Multicellularity is the next frontier of synthetic biology!
It is our vision that living multicell systems will become as programmable, interactive, constructible, and useful as our personal electronic devices.
Our current research focuses on engineering multicellular bacterial systems while controlling their morphology, patterning, dynamics, and environmental responses. We combine biophysics, synthetic biology, mathematics, and interaction design. We recently engineered the first synthetic, fully genetically encoded cell-cell adhesion toolbox (Glass Cell 2018) and the first optogenetic high-resolution cell-surface patterning method (‘Biofilm Lithography’) (Jin PNAS 2018) – providing key missing tools for engineering synthetic multicell systems (Kim Nature 2022). We also pioneered the field of Interactive Bio-Technology (IBT) that enables humans to directly interact with and program living multicell assemblies in real-time (Lee Frontiers in Computer Science 2022).
Motivating research questions:
- What are the rules of bacterial self-assembly of complex 3D morphologies and patterns?
- How does antibiotic resistance emerge in multi-species biofilms?
- Can we modularize complex metabolic pathways among multiple cells, e.g., for drug synthesis, bioremediation, and greenhouse gas reduction?
- How to engineer synthetic developmental programs?
- Are microbiologists really that small?
- Can we create environmentally responsive smart materials?
- What (digital?) user interface are required for programming of and interacting with such systems?
- Can we uncover new laws regarding the physics of active matter?
- Can we understand the evolutionary transition to multicellular life (on earth and beyond)?
If you would like to join the lab or collaborate – contact us!
Prior Research Achievements
Synthetic adhesins, biofilm-lithography, self-assembly, adhesion logic, multicellular patterning
The next frontier of synthetic biology concerns multicell systems, e.g., to design novel materials, to engineer complex multi-component metabolic pathways, and to understand multicellular evolution and development. Such efforts require tools to adhere cells into defined morphologies and patterns. We engineered the first 100% genetically encoded synthetic platform for modular cell-cell adhesion based on orthogonal membrane-displayed nanobody-antigen pairs. We engineered an optogenetic method (“Biofilm Lithography”) that allows the deposition of E. coli with blue light at high spatial resolution (down to 25 µm). We demonstrated the rational design of various bacterial self-assembly morphologies and pattern. We developed a synthetic 4-bit adhesion logic for universal interface patterning in 2D systems. These toolboxes are fully compatible with synthetic biology standards, enable control over adhesin affinity and strength, and will enable the future design of complex high-level multicellular systems for above-mentioned applications.
4-bit adhesion logic enables universal multicellular interface patterning |
|
A genetically encoded adhesin toolbox for programming multicellular morphologies and patterns |
|
Biofilm Lithography enables high-resolution cell patterning via optogenetic adhesin expression Image
![]() |
Multicell patterning dynamics in developmental systems
The coordination of patterning and growth during development in natural multicellular systems poses many intriguing questions. We studied theoretically and experimentally various multicellular systems where intercellular delta-notch signaling coordinates the transient differentiation among large number of cells. We found that depending on signaling strength, signaling delays, and developmental noise different dynamic patterns can be achieved, e.g., synchronized oscillations, traveling waves, or lateral inhibition patterns, furthermore that critical parameter conditions exist beyond which error-free multicellular patterning can be guaranteed.
Nonlinear delay differential equations and their application to modeling biological networks |
|
Stem Cell Scaling during Adult Organ Resizing |
|
Signaling Delays preclude Defects in Lateral Inhibition Patterning |
|
Synchrony dynamics during initiation, failure and rescue of the segmentation clock. Image
![]() |
Microswimmers: Molecular motors, flagellar beating, taxis, collective behaviors
Single celled microswimmers exhibit versatile strategies for sensing and navigating their environment. They are often driven by cilia (eukaryotic flagella), i.e., slender cellular appendages whose regular beating propels such cells through aqueous media. The beat is an oscillating pattern of propagating bends generated by dynein motor proteins. A key open question is how the activity of these molecular motors is coordinated in space and time. Using theory and experiments, we analyzed the beating behavior sperm flagella with high precision. We determined how the flagellar beat patterns are regulated through an interplay of basal properties of the axoneme and the mechanical feedback of dynein motors; furthermore, how stochastic dynamics of individual motors give rise to active small-number fluctuations in motor-cytoskeleton systems. We also studied individual and collective behaviors such microswimmers. Among others, we discovered the self-organization of sperm cells into vortex arrays, and the polygonal swimming patterns of Euglena gracilis. Experimental-theoretical biophysical analysis of these striking phenomena revealed functional links between molecular sensors and actuators, simple cellular control-feedback loops, and long-term task achievement, e.g., for efficient chemo- and phototaxis through complex, ecologically relevant stimulus fields.
Polygonal motion and adaptable phototaxis via flagellar beat switching in the microswimmer Euglena gracilis |
|
A self-organized vortex array of hydrodynamically entrained sperm cells. |
|
How molecular motors shape the flagellar beat. |
|
Active Phase and Amplitude Fluctuations of Flagellar Beating Image
![]() |
Programming of collective microswimmer behaviors and swarm robotics
Controlled swarms of active microscopic agents have many potential applications, e.g., biomedical and environmental diagnostics, on-chip transport, signal transduction, or self-assembly of active materials and microdevices. However, programming algorithms and user interfaces for the efficient control of such swarms are lacking. We conceptualized and implemented corresponding swarm programming paradigms that provide stimulus and sensor control functions for real-time manipulation of collective active matter system. Future applications include the integration with synthetic microswimmers to control multicell bacterial self-assembly and actuation (see above).
Interactive programming paradigm for real-time experimentation with remote living matter |
|
Device and Programming Abstractions for Spatiotemporal Control of Active Micro-Particle Swarms Image
![]() |
Interactive bio-technology and biotic games
Computer technology has evolved from large number-crunching machines in the 1950s to today’s interactive devices of ubiquitous professional and personal use. We are inspired by people like Douglas Engelbart, Joseph Licklider, Ralph Baer, and Seymour Papert, who made computing interactive, accessible, intuitive, affordable, and fun. To enable equivalent impact for the life-sciences, we pioneered the human-interaction design and application space that is provided by techniques like microfluidics, lab robotics, and genetic engineering - which we term Interactive Bio-Technology (IBT). We first demonstrated this paradigm through ‘biotic games’, where a human can play with living cells in realtime. This represents an entirely new game type which merges wet biology with video games. Thinking more broadly, this new interactive medium has many potential affordances, e.g., lowered access barriers to research instruments, new hands-on microfabrication approaches, novel educational technology, or improved usability for medical devices.
Scientific Discovery Games for Biomedical Research. |
|
Interactive Biotechnology: Design Rules for Integrating Living Matter into Digital Games |
|
A Biotic Game Design Course |
|
Innocent fun or microslavery?: Public Responses to and Ethical Analysis of Biotic Games |
|
Design, Engineering and Utility of Biotic Games Image
![]() |
Interactive biology cloud experimentation labs for research and education
Biology cloud experimentation labs, akin to the cloud computation paradigm, promise collaborative time-sharing of and lowered access barriers to life-science instruments for academia, industry, and education. We conceptualized and implemented asynchronous and synchronous biology cloud labs for interactive experimentation. Our synchronous cloud lab architecture enables millions of biology experiment/year at <1 cent/experiment. Such performance is possible when adapting the cloud computing paradigm to the life-sciences with ubiquitous, on-demand access to a shared pool of configurable, hot-swappable, and distributed experimentation resources, i.e., a scalable cluster of Biotic Processing Units (BPUs). Biology cloud labs have many applications, e.g., for professional and citizen science, or science education (see below), with wider adaptation expected as life-science automation advances in flexibility, price, and throughput. Cloud lab technology promises global impact on classic on-site as well as (massive open) online STEM education. We evaluated our cloud lab with over 700 participants in various settings by now, e.g., middle and high-school, college, and as open edX online course also reaching developing countries. Users were enabled to execute key scientific practices (open-ended and controlled experimentation, data collection, visual and quantitative data analysis, biophysical modeling – ending with self-initiated projects including discovery and hypothesis testing). This represents a paradigm shift of what students currently can do in either on-site or online life-science education, i.e., going from passively observing cells through a microscope to truly interacting with them. This fills an unmet need for novel, deep inquiry approaches in STEM education as voiced by many national science standards.
Design Guidelines and Empirical Case Study for Scaling Authentic Inquiry-based Science Learning via Open Online Courses and Interactive Biology Cloud Labs |
|
Life-Science Experiments Online: Technological Frameworks and Educational Use Cases |
|
Interactive and Scalable Biology Cloud Experimentation for Scientific Inquiry and Education |
|
Cloud Experimentation for Biology: Systems Architecture and Utility for Online Education and Research Image
![]() |
Informal and formal STEM education
We collaborate with educational experts to evaluate the real-world potential of interactive bio-technology for formal and informal science education: (i) A museum exhibit (TrapIt!) enables to interrogate living cells with light via a touchscreen, which is installed as long-term exhibit for multiple years now in the Exploratorium (San Francisco Science Museum - approx. 1 million annual visitors); (ii) A smartphone microscope (LudusScope) combines construction, play, and inquiry; (iii) Low-cost Lego Mindstorm pipetting robots with research grade performance bridge mechatronics and wet-science education; (iv) Biology cloud labs that can reach millions of learners (see above).
Pac-Euglena: A living cellular Pac-Man meets virtual ghosts |
|
First-hand, immersive full-body experiences with living cells through interactive museum exhibits |
|
Classroom on a Chip: Taking Microfluidics from the Laboratory into the Classroom |
|
LudusScope: Accessible Interactive Smartphone Microscopy for Life-Science Education |
|
Liquid-handling Lego robots and experiments for STEM education and research |
|
Trap it! : A Playful Human-Biology Interaction for a Museum Installation Image
![]() |